Sandbox Lab: Visualizing Fluid Flow
2023 Sandbox Lab Results & Beyond
Researchers: Dr. Hailun Ni, Dr. Tip Meckel,
David DiCarlo and M.S. student Jose Ubillus
2018–2022 Sandbox Lab Background
Researchers: Drs. Prasanna Krishnamurthy,
Luca Trevisan, Tip Meckel, and David DiCarlo
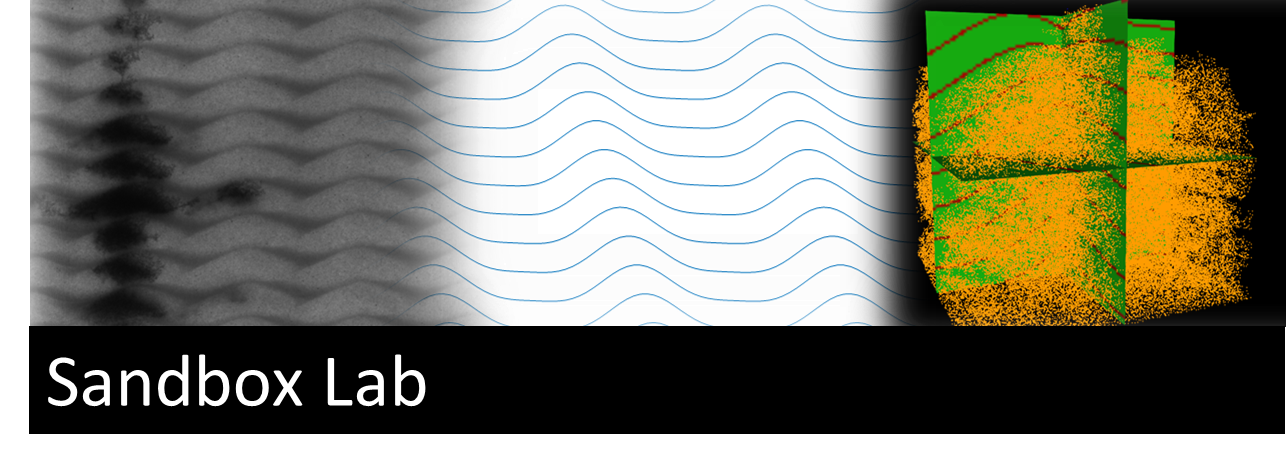
About the Sandbox Lab
In order to decrease greenhouse gas emissions, carbon dioxide (CO2) may be captured, transported, and injected into rocks deep below the ground surface (> 0.8 km; what we often refer to as the subsurface). Seeing the fine details of what happens to CO2 a half mile or more beneath the Earth’s surface is difficult to observe firsthand. Since 2018, scientists at the Gulf Coast Carbon Center (GCCC), have been recreating subsurface geologic conditions (i.e., heterogeneities) in a laboratory setting in order to model how CO2 will move and disperse in rock layers deep below the ground surface after CO2 is injected.
When CO2 is injected, and stored in the subsurface, it exists in a phase known as a supercritical fluid. Supercritical CO2 has a density similar to liquid but has a viscosity similar to a gas. GCCC researchers have begun to understand how different rock attributes affect how CO2 flows as a fluid in the subsurface and are currently modeling how CO2 is moving in the subsurface.
Our Story
The research effort that led to the development of the sand tank lab started, like many research projects, with an idea: How can we better characterize and quantify CO2 flow in heterogeneous clastic rocks? Since heterogeneity is a traditionally challenging research topic in geology, the intent was to have a departure point for this research that was different than prior work but supported by it.
The first effort involved the use of a sedimentary relief lacquer peel, which was a method of research pioneered in the 1960's to study modern depositional environments. Dr. Tip Meckel happened to have several of these from Shell's Bellaire Research Lab in Houston. So, Tip took one that hangs on his office wall representing the uppermost portion of a point bar deposit from the Brazos River, and produced this research paper:
Meckel, T.A., 2013, Digital rendering of sedimentary-relief peels: Implications for clastic facies characterization and fluid flow: Journal of Sedimentary Research, v. 83, no. 6, p. 495–501, http://dx.doi.org/10.2110/jsr.2013.43
Encouraged by those results, Tip next considered how to incorporate realistic sedimentary heterogeneity in three dimensions (3D), and immediately recalled the excellent models developed by David Rubin at USGS. Tip studied these images in Dr. Gary Kocurek's sedimentology class as a graduate student at UT-Austin, and he was keen to develop usable models of these fairly realistic bedforms for fluid flow simulation. Luckily, Carissa Carter had converted Rubin's original codes into MATLAB. After many months understanding how the code works, Tip was able to modify the code to generate 3D models of laminae and matrix. After testing ideas in two dimensions, it was straight-forward to assign threshold pressures related to grain sizes and simulate buoyant fluid flow. That work was led by post-doc Luca Trevisan and Ph.D. student Prasanna Krishnamurthy, and is summarized in this publication. The methods and codes are available.
Luca had amazing experience based on his excellent dissertation at Colorado School of Mines, and we extended that effort to include numerical simulation. Prasanna's dissertation research was co-supervised by Dr. David DiCarlo and Dr. Tip Meckel. The success of the sand tank lab development is largely due to their tireless efforts. Prasanna saw that there was a way to modify the original work published by Clark Griffith, Robert Holt and Bob Glass by adding a more sophisticated linear actuator that could be programmed to deposit sand mixtures in a very repeatable way—his dissertation—is a master work. Through this effort, we realized that there was so much that could be investigated methodically, so we hired the brilliant Dr. Hailun Ni after her PhD at Stanford with Dr. Sally Benson to carry the sand tank lab work forward as a post-doctoral fellow. Despite an ongoing pandemic, she worked remotely with Prasanna and learned how to operate the lab. They recorded their many conversations, which should be a great resource for others who want to get into this kind of research. Hailun stayed on as a Research Associate at the Gulf Coast Carbon Center, and her work led to a series of publications on various aspects of the topic. She now supervises excellent students, please contact her if you are interested in pursuing a project.
A side-trip on this heterogeneity and fluid flow journey was taken with the brilliant Emily Beckham, now Emily Wood at ExxonMobil. Her M.S. thesis was a very ambitious effort to use a previously generated sand tank model of a delta at Chris Paola's amazing lab at the University of Minnesota to build a digital reservoir model and simulate flow from CO2 injection. Read more about this research here.
What's next?
Want to be a part of it?
Get in touch with Dr. Hailun Ni.
Select Publications
Ni, H., Bakhshian, S., Meckel, T.A., 2023, Effects of grain size and small-scale bedform architecture on CO2 saturation from buoyancy-driven flow: Scientific Reports, v. 13, p. 1–13, https://doi.org/10.1038/s41598-023-29360-y
Krishnamurthy, P.G., DiCarlo, D., and Meckel, T.A., 2022, Geologic heterogeneity controls on trapping and migration of CO2: Geophysical Research Letters, American Geophysical Union, 49, p. 19, https://doi.org/10.1029/2022GL099104.
Ni, H., Braganca, R., Tisato, N., Meckel, T.A., 2022, Monitoring CO2 plume migration with lab-scale ultrasonic experimental setup (paper), 16th Greenhouse Gas Control Technologies Conference Lyon, 23–27 October, 2022 (GHGT-16), 9 p., http://dx.doi.org/10.2139/ssrn.4273218.
Ni, H., Meckel, T.A., 2022, Effects of flow pulsation on CO2 buoyant migration and capillary trapping (paper), 16th Greenhouse Gas Control Technologies Conference Lyon, 23–27 October 2022 (GHGT-16), 9 p., http://dx.doi.org/10.2139/ssrn.4273191.
Bump, A.P., Bakhshian, S., Ni, H., Hovorka, S., Dunlap, D., Olariu, M., Hosseini,S., Meckel, T., 2022, Composite confining systems: Characterizing, de-risking and permitting unconventional seals for CO2 Storage (paper), 16th Greenhouse Gas Control Technologies Conference Lyon, 23–27 October, 2022 (GHGT-16), 11 p., http://dx.doi.org/10.2139/ssrn.4286411.
Ni, H., and Meckel, T. A., 2021, Characterizing the effect of capillary heterogeneity on multiphase flow pulsation in an intermediatescale beadpack experiment using time series clustering and frequency analysis: Water Resources Research, v. 57, no. 11, 17 p., http://doi.org/10.1029/2021WR030876.
Krishnamurthy, P.G., 2020, Geologic heterogeneity controls on CO2 migration and trapping [Dissertation], The University of Texas at Austin, Austin, Texas, 194 p., http://dx.doi.org/10.26153/tsw/11921
Krishnamurthy, P.G., Meckel, T.A., and DiCarlo, D., 2019, Mimicking geologic depositional fabrics for multiphase flow experiments: Water Resources Research, v. 55 no. 11, p. 9623–9638, https://doi.org/10.1029/2019WR025664
Trevisan, L., Krishnamurthy, P.G., and Meckel, T.A., 2017, Impact of 3D capillary heterogeneity and bedform architecture at the sub-meter scale on CO2 saturation for buoyant flow in clastic aquifers: International Journal of Greenhouse Gas Control, v. 56, p. 237–249, https://doi.org/10.1016/j.ijggc.2016.12.001
Student Products
Krishnamurthy, P. G., 2020, Geologic Heterogeneity Controls on CO2 Migration and Trapping: PhD Dissertation, The University of Texas at Austin, Austin, Texas, 213 p.
Ubillus, J. E., 2024, Laboratory Experiments and Modeling to Evaluate Critical CO2 Saturation for Geologic Carbon Storage: M.S. Thesis, The University of Texas at Austin, Austin, Texas, 59 p.
Last updated: July 11, 2024